
Steven Weinberg's four tips for aspiring scientists

8 times when Nikola Tesla was wrong about physics

Nikola Tesla was a great Serbian-American engineer who played the major role in perfecting and promoting alternate current. He was also a visionary who predicted smartphones, renewable energy and creation of artificial Suns, much before time permitted.
However, it is surprising that Tesla sometimes took anti-science as well as anti-mathematics positions. Several of his views about the world were particularly pseudoscientific. So in this post, let us look at 8 instances when even the Genius Nikola Tesla was wrong.
On electrons
Tesla did not agree with the theory of atoms being composed of smaller subatomic particles. He thought that there was no such thing as an electron creating an electric charge and that it had nothing to do with electricity.
However, not only did the electron get discovered but also its properties and effects were measured by physicist J.J. Thomson at the start of the twentieth century. Without electron, technologies like the television couldn't exist.
On relativity
According to Nikola Tesla, Einstein's 1915 theory of general relativity was wrong. He commented in 1932: "I hold that space cannot be curved, for the simple reason that it can have no properties. It might as well be said that God has properties. He has not."

In 1935, Tesla told The New York times: "Einstein's relativity work is a magnificent mathematical garb which fascinates, dazzles and makes people blind to the underlying errors. The theory is like a beggar clothed in purple whom ignorant people take for a king."
In 2004, the gravity probe-b satellite was launched to measure the curvature due to Earth. Its data was analyzed by the Stanford University and it indeed confirmed Einstein's theory to a high degree of accuracy in 2011.
Furthermore, without relativity, the GPS would fail in its navigational functions and Google maps couldn't work to pinpoint precision.
On mathematics
Nikola Tesla said in 1934: "Today's scientists have substituted mathematics for experiments, and they wander off through equation after equation and eventually build a structure which has no relation to reality."
That may be true, although mathematics and experiments are both fundamental to scientific progress. There cannot be one without the other, especially in the field of physics.
At the same time in Europe, Dirac was trying to find an equation to unify quantum mechanics and special relativity. He predicted the existence of antimatter in doing so, which was discovered in 1932.
Even 16th century Galileo Galilei had a high regard for mathematics, when he said: Philosophy is written in mathematical language; without it one wanders in vain through a dark labyrinth.
On atomic energy
Tesla told The New York Times in 1931: "The idea of atomic energy is illusionary. I have preached against it for twenty-five years but there are still some who believe it to be realizable."
Because, as mentioned before, he did not trust the theory of subatomic particles. So according to Tesla, atoms were immutable – meaning that they could not be split or changed in any way.
Two years after Tesla's death in 1943, not only did the humankind split the atom, they also used it to end the World War II. Although it began a nuclear arms race and a call for disarmament – well that is another story in itself.
Today, atomic energy is a source of nuclear power – as predicted by physicist Lise Meitner – which is in turn used to generate heat and electricity. Moreover, scientists are also working on a large-scale fusion project called ITER for future electricity generation.
On EM waves
German physicist Henrich Hertz demonstrated the accuracy of Maxwell's equations when he successfully generated electromagnetic waves in laboratory.
Because Tesla did not have the mathematical advantage, he relied completely on experiments and his own experiments led him to erroneously believe that Hertz and Maxwell were wrong.
In one 1891 lecture, Tesla expressed openly his disagreements with Hertz – which is anyway healthy for the sake of scientific progress.
But over the next few years, several groundbreaking evidences were collected in the favor of Maxwellian electromagnetism.
In 1898, Tesla himself developed a radio based remote-controlled boat and yet till 1919 he did not believe in the existence of EM waves and in the theories developed by Maxwell and Hertz.
On wireless electricity
Tesla was a great visionary but his vision was not always practical. After perfecting alternate current technologies, Tesla wanted to make a new revolutionary change - render wires useless!
At first, Tesla decided to transmit electricity through air but rejected the idea later on. In 1902, Tesla completed the Wardenclyffe Tower to tranfer electricity via ground.
However, engineers pointed out that currents once injected into the ground would spread in all the directions, quickly becoming too diffuse to be usable over long distances.

In addition to engineering and financial problems, the dangers of wireless electrical power to nearby wildlife was not taken into account by Tesla. Thus, the Wardenclyffe Tower project had to be abandoned.
During the same time, Italian engineer Guglielmo Marconi - who unlike Tesla, believed in and worked with electromagnetic waves, succeeded in the wireless transmission of information, rather than electricity.
On science
Although Nikola Tesla was a brilliant engineer and inventor, he sometimes delved into pseudoscientific ideas which had no basis in reality and lacked experimental data – a quality he admired.
For example, Tesla once said: A single ray of light from a distant star falling upon the eye of a tyrant in bygone times may have altered the course of his life, may have changed the destiny of nations.
That thought, although poetically is beautiful, has no scientific weight. Distant stars and planets and their motions have no measurable effects on people. What changes destiny of nations is politics and the king's advisor would have had far greater impact than light of a far away star.
On radioactivity
In 1903, Marie Curie, Pierre Curie and Henri Becquerel won the Nobel Prize in physics for discovering evidence for radioactivity.
However, Tesla was not convinced since he did not believe that the atom was divisible and that it had internal forces and subatomic particles.
According to him, the phenomena of radioactivity was not the result of forces within the radioactive substance but by the rays emitted by the Sun.
He told The New York Times in 1931: If radium could be screened effectively against this ray it would cease to be radioactive.
Summing up
Nikola Tesla was a genius inventor and explorer whose work ushered the electrical revolution that transformed daily life. Einstein wrote to Tesla: As an eminent pioneer in the realm of high frequency currents... I congratulate you on the great successes of your life's work.

But at the same time Nikola Tesla was also human – jealousy, denial and frustration, played a big role in his professional life.
His frustration with advanced mathematics led him to incorrectly conclude that Maxwell's equations and relativity were wrong.
His denial of modern science left him too far behind his contemporaries – Marconi, Braun, Bose – in his ability to contribute to the wireless communication.
Surely, Tesla did achieve what others could only dream of. But the point is, not to put Tesla on pedestal, or build conspiracy theories in his favor, as many fans would want to do. It does not do justice to Tesla's brilliance.
10 TV Shows That Physics Students Will Enjoy

1. Steins; Gate
2. Big Bang Theory
3. Star Trek

4. Doctor Who

5. Young Sheldon
6. Dr. Stone
7. Black Mirror
8. Rick and Morty
9. Battlestar Galactica
10. The Expanse
The Expanse which you can watch on Amazon Prime is a beautiful combination of space engineering and fiction. It has some of the best physics-based spaceflight and combat and an engaging story as well, according to one viewer.
10 Nobel Prize Winning Families In Science

Curie family
Niels and Aage Bohr
Raman and beyond
Thomson family
Arthur and Roger Kornberg
Euler family
Manne and Kai Siegbahn
Bragg family
May-Britt Moser and Edvard Moser
Carl Ferdinand and Gerty Cori
7 Lessons To Learn From Richard Feynman
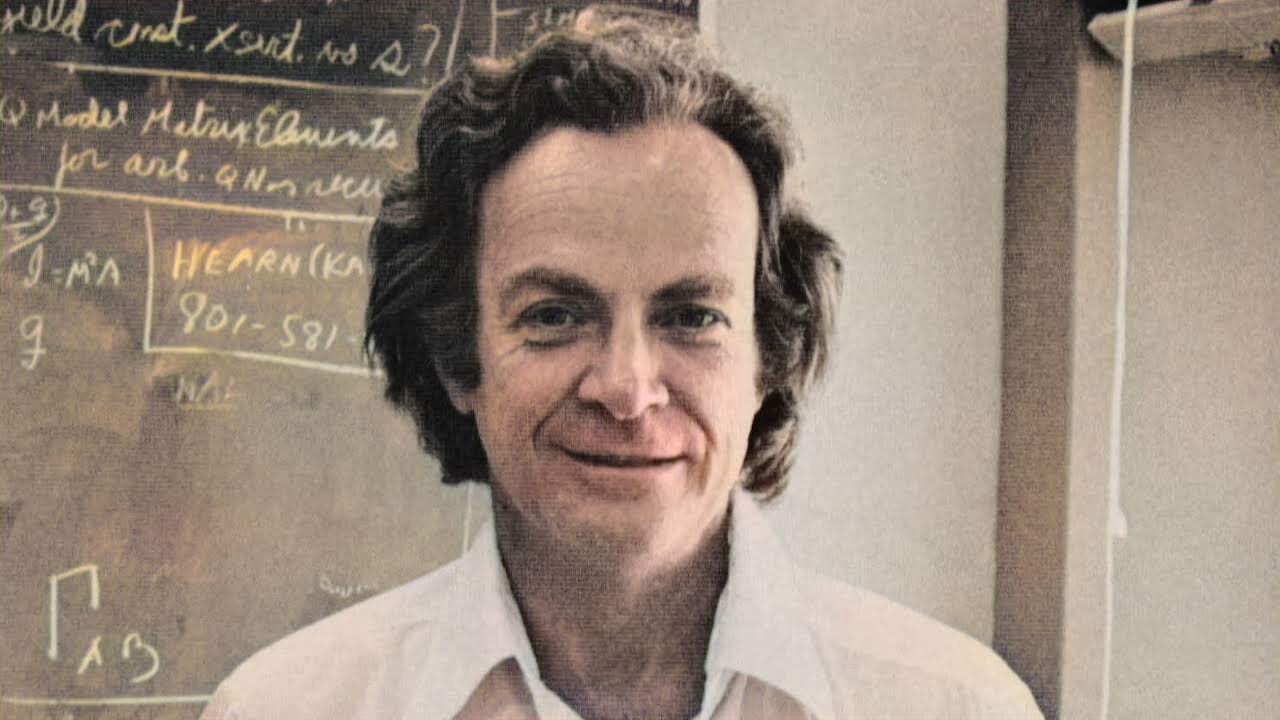
1. Pursue a hobby
2. Explore the world
3. Carve your own path
4. Keep learning
5. You only live once
6. Blind belief is dangerous
7. Enjoy the process
Top 10 Important Equations In Physics


It tells you how powerful an engine has to be in order to pull a car, how much thrust required to lift a rocket, how far a cannonball flies and so on. But more importantly, the equation helped debunk the Aristotelian beliefs which had remained unchallenged for thousands of years.
According to Aristotle, force is necessary to keep an object going. Why otherwise would a ball rolling on the ground eventually stop? Because, Aristotle said, it isn't pushed anymore, that's why.
Then, in the 17th century, Italian scientist Galileo Galilei explained, with experiment, "The ball stopped due to the ground being rough and had it been sufficiently smooth, the ball would roll forever. No force required!"
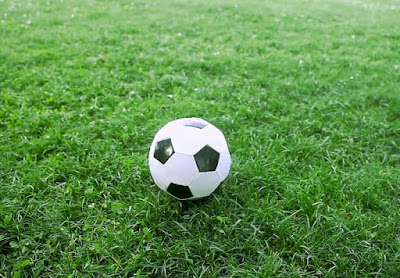
Newton said further, that the state of rest or uniform linear motion both imply zero acceleration. Thus, the particle will remain in place or keep going at the same rate and it will maintain itself in the state in which it's been until of course when acted upon by external force.
In its most familiar form, it says that the more precise the measurement of position, the more imprecise the measurement of momentum, and vice-versa. Thus, one can never know with perfect accuracy both of those two important factors which determine the movement of one of the smallest particles, its position and its velocity, at the same instant.

The uncertainty principle was immediately rejected by leading physicists of the time, including Albert Einstein. There, Niels Bohr did try his best to convince Einstein that the uncertainty relation is fundamental law in physics. Einstein still refused, and they agreed to disagree. By 1933, the political situation became much worse in Germany, and Einstein moved to the United States.
In 1954, Heisenberg visited Einstein's house in Princeton. They talked only about physics, but Einstein's position on the principle hadn't changed. In 1955, Einstein passed away leaving Werner Heisenberg disheartened that he had failed to get Einstein's endorsement of his uncertainty relation.
Although Einstein and others objected to Heisenberg's and Bohr's views, even Einstein had to admit that they were indeed a logical consequence of quantum mechanics. But for Einstein, something still was missing and the quantum mechanics was incomplete, "I am convinced that god does not throw dice," he claimed metaphorically.
Heisenberg, supported by Bohr, Pauli, Schrödinger and others, maintained until his death that quantum uncertainty is not inaccuracy of the measurement, it is inherent in quantum phenomena. It leads to probabilistic and not deterministic outcomes.

Generators and motors both make use of Faraday's Law. The equation by Maxwell became the foundation of power generation hence making Faraday the father of electricity. Maxwell said of Faraday, "He is, and must always remain, the father of that enlarged science of electromagnetism."
Symmetry is the keyword of physics and Dirac used it perfectly in 1928. He developed an equation that explained spin number as a consequence of the union of quantum mechanics and special relativity. The equation also predicted the existence of anti-matter, previously unsuspected and unobserved, and which was experimentally discovered in 1932.

This accomplishment has been described on par with the works of Newton, Maxwell, and Einstein before him. Dirac even speculated that there may also be mirror universe of anti-particles, thus becoming a source of inspiration for science-fiction writers. Dirac was also equally famous for his contribution to quantum electrodynamics, which described how electric and magnetic forces would work on the scale of things smaller than atoms.
The famous inequality which says that when energy changes from one form to another form, or when matter moves freely, the disorder in a closed system increases. According to renowned astronomer Arthur Eddington, "The law that entropy always increases, holds, I think, the supreme position among the laws of nature."
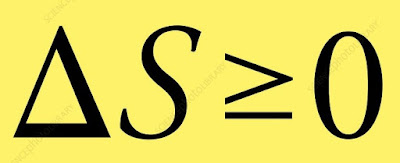
The concept of the second law of thermodynamics applies not only to internal combustion engines used in our cars, motorcycles, ships and airplanes but also to explain the processes of life, when considered in terms of cyclic processes.
The second law also has profound consequences for the universe in large scale. Imagine being shown a video clip of a cup being dropped and breaking. You'd clearly be able to tell whether the video was being played backward or forward, from the flow of entropy.
Similarly, if the movie of our universe is played backwards, the universe would be getting more and more ordered, like the cup, and when played forward, we'd expect it getting disordered, like the pieces of broken cup.
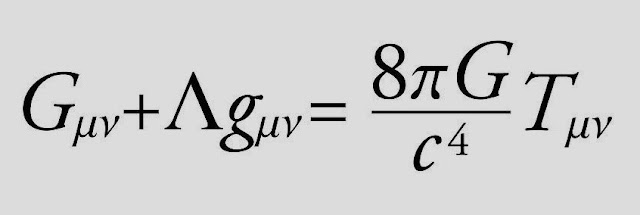
Einstein's equations led to the fusion of the three dimensions of space and the one dimension of time into a single four-dimensional spacetime. The expression on the left hand side of the equation represents the curvature of spacetime. The expression on the right is the energy density of spacetime. The equation dictates how energy determines the curvature of space and time.
The cosmological constant term (Λ) was introduced by Einstein to allow for a universe that is not expanding or contracting. This effort was unsuccessful because in 1929, astronomer Edwin Hubble discovered evidences for an expanding universe. Einstein was invited by Hubble to see for himself that the universe indeed was changing.

As a result, Einstein abandoned the cosmological constant in the equation, calling it the biggest blunder he ever made. So from the 1930s until the late 1990s, most physicists assumed the cosmological constant to be equal to zero. But, recently improved astronomy techniques have found that the expansion of the universe is accelerating implying the non-zero value of the constant.
Why are the Einstein field equations important in physics? Firstly, because they unify the two concepts of space and time, previously considered separate by the limitations of our intuition, into one spacetime. Just like Maxwell had unified electricity and magnetism into electromagnetism in the 19th century.
Secondly, they describe – not the force – but the fundamental "interaction" of gravitation as a result of spacetime being curved by energy (mass too is energy from Einstein's energy-mass equivalence).
Although Newton did give the formula to calculate the magnitude of gravitational force between any two bodies of mass separated by a distance, he didn't quite explain the cause of gravitation in the first place.

The single-dimensional wave equation has a scalar function (u) of one space variable and one time variable since waves propagate in space, and in time also. This equation was first written by French mathematician Jean le Rond d'Alembert, hence it's sometimes also called the d'Alembert's equation. Swiss mathematician and physicist Leonhard Euler wrote it in three dimensions in 1707.
We are constantly surrounded by waves, whether perceptible to us or not, they are always there. Like when you play a guitar or drop a stone into a pond. The wave equation isn't as elegant as others on this list but it is groundbreaking as it's been applied to sound waves (and instruments), waves in fluids, waves in earthquakes, light waves, quantum mechanics and general relativity.
This formula is responsible for the birth of quantum mechanics, also television and solar cells. Leading German physicist of the time Max Planck postulated in 1900, that energy was quantised and could be emitted or absorbed only in integral multiples of a small unit, which he called "energy quantum".

Einstein extended Planck's idea in 1905 when he introduced the concept of "light quantum", the particle of light, or photon. Thus, the electromagnetic radiation wasn't continuous like a wave but isolated in the packets of light, Einstein proposed.
Planck had simply introduced the equation as a trick to solve a problem with black body radiation, but Einstein envisioned it to be more. In 1887, experimenter Heinrich Hertz stumbled upon the photoelectric effect for the first time; the emission of electrons when light of specific frequency hit a material.
The phenomenon of photoelectric effect remained largely unexplained, even with the wave theory of light, until the arrival of Planck-Einstein relation in 1905. Einstein described it in terms of particle-particle interaction between the photon and electron. He said, "...below some critical frequency, no photon has enough energy to knock an electron free."
This means that if a photosensitive material requires photons of blue light to emit the electrons, which is the characteristic of the material, then the photons of green or yellow light won't be able to knock the electrons out of the material.

The characteristic energy or work-function of the material is absorbed, to loosen the bonds, and then the remainder of the energy is observed as kinetic energy of the free electron. Einstein's clarification was consistent with the law of conservation of energy. He was recognized with Nobel Prize in physics for his explanation of the photoelectric effect (and not for energy-mass relation or relativity).
Planck said his introduction of "quantum" in 1900 was an act of desperation but when Einstein adopted it and gave it meaning, a whole new debate had started and the old laws were swept away within a decade or so. Einstein who himself was accountable for it refused to endorse the new quantum revolution.
The discovery by Planck and Einstein became the basis of all twentieth-century physics, without which, it would not have been possible to establish a workable theory of molecules and atoms and the energy processes that govern their transformations.

Just like Newton's equations are used to calculate how a football behaves when kicked, you use the Schrödinger's equation to calculate the behaviour of electron in the orbit of an atom. More generally, it is used for many calculations in quantum mechanics and is also fundamental to much of the modern technology, from lasers to transistors, and the future development of quantum computers.